
Maybe. Water utilities are now testing for this possibility. The truth is not yet known completely regarding this issue.
But here's what is known so far:
Water utilities measure arsenic at the entry point to a distribution system for compliance determinations. When the arsenic level in the treated water supply is below the 10 parts per billion drinking water standard, that particular drinking water source is generally considered safe for human consumption at the tap. Just what is arsenic and how did it get near our drinking waters?
Arsenic is released to the environment from a variety of natural and anthropogenic sources. In the environment, arsenic occurs in rocks, soil, water, air, and biota. Average concentrations in the earth's crust reportedly range from 1.5 to 5 mg/kg (Cullen and Reimer, 1989). Higher concentrations are found in some igneous and sedimentary rocks, particularly in iron and manganese ores (Welch et al., 1988). In addition, a variety of common minerals contain arsenic, of which the most important are arsenopyrite (FeAsS), realgar (AsS), and orpiment (As2S3). Natural concentrations of arsenic in soil typically range from 0.1 to 40 mg/kg, with an average concentration of 5 to 6 mg/kg (National Academy of Sciences (NAS), 1977). Through erosion, dissolution, and weathering, arsenic can be released to groundwater or surface water. Geothermal waters can be sources of arsenic in groundwater, particularly in the Western United States (Nimick et al., 1998, Welch et al., 1988).
Other natural sources include volcanism and forest fires. Anthropogenic sources of arsenic relate to its use in the lumber, agriculture, livestock, and general industries. Most agricultural uses of arsenic are banned in the United States. However, organic arsenic is a constituent of the organic herbicides monosodium methanearsonate (MSMA) and disodium methanearsonate (DSMA), which are currently applied to cotton fields as herbicides (Jordan et al., 1997). Organic arsenic is also a constituent of feed additives for poultry and swine and appears to concentrate in the resultant animal wastes (NAS, 1977).
The potential impact of arsenic in animal wastes used to fertilize crops is uncertain. Most of the arsenic used in the United States is for the production of chromate copper arsenate (CCA), the wood preservative (Reese, 1998). CCA is used to pressure treat lumber and is classified as a restricted-use pesticide by the USEPA. Significant industrial use of arsenic is the production of lead-acid batteries, while small amounts of very pure arsenic metal are used to produce the semiconductor crystalline gallium arsenide, which is used in computers and other electronic applications.
Arsenic is also released from industrial processes, including the burning of fuels and wastes, mining and smelting, pulp and paper production, glass manufacturing, and cement manufacturing (USEPA, 1998b). In addition, past waste disposal sites may be contaminated with arsenic. Arsenic is a contaminant of concern at 916 of the 1,467 sites on the National Priorities List (NPL) (Agency for Toxic Substances and Disease Registry (ATSDR), 1998). Sites included on the NPL have the potential to release contaminants to groundwater or surface water in the vicinity of the site. Anthropogenic releases of arsenic to the environment can be estimated from Toxics Release Inventory (TRI) data. These data indicate that 7,947,012 pounds of arsenic and arsenic-containing compounds were released to the environment in 1997, a significant increase from 3,536,467 pounds in 1995 (USEPA, 1999a). The increase primarily occurred at one facility, where arsenic on-site land releases increased by 3.58 million pounds from 1995 to 1997 because of a change in the facility's smelting process that was implemented to reduce sulfur dioxide emissions.
The TRI data do omit some potentially significant arsenic sources, including arsenic associated with the application of herbicides and fertilizers and arsenic released from mining facilities and electric utilities. Arsenic Fate and Transport Once arsenic released from natural or anthropogenic sources enters groundwater or surface water, a variety of processes affect its fate and transport. These include oxidation-reduction reactions, transformations, ligand exchange, and biotransformations. The factors that affect these reactions include the oxidation state of the arsenic, oxidation-reduction potential (Eh), pH, concentrations of iron, metal sulfides, and sulfides, temperature, salinity, and the distribution and composition of biota (ATSDR, 1998; Robertson, 1989; Welch et al., 1988). The predominant forms of arsenic in groundwater and surface water are arsenate (+5) and arsenite (+3). Arsenite is generally associated with anaerobic conditions. Oxidation state appears to be the most important factor that determines the fate and transport of arsenic through drinking water treatment systems. Arsenate is more easily removed because of its ionic charge, and activated alumina, ion exchange, and reverse osmosis technologies can achieve relatively high arsenic removal rates.
These technologies do not achieve comparable removal rates for arsenite. Oxidization of arsenite to arsenate can improve removal efficiencies. Treatment efficiencies may also be affected by water pH, depending on the technology applied, and competing ions. Higher pH tends to decrease removal rates (Rubel and Hathaway, 1987); high sulfate, fluoride, and phosphate concentrations also tend to decrease removal rates (Jekel, 1994).
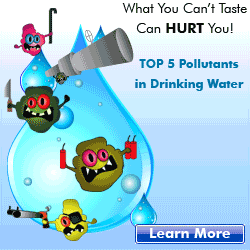
However, it has now been shown that arsenic residual in treated water can become attached to iron components in distribution systems to form relatively arsenic-rich scales. Under conditions of increased flow or changes in water chemistry, these arsenic-rich scales can become dislodged or the arsenic within them can become re-suspended in the water, and thereby, ultimately delivered to consumers' taps.
There are a variety of sources of information on arsenic scales in drinking water. This study is based largely on arsenic data from 25 State compliance monitoring data sets, and information on individual system characteristics that are provided in the Safe Drinking Water Information System (SDWIS). Figure ES-1 presents the States for which compliance monitoring data were available. As this figure shows, the Midwestern, South Central, North Central, and Western regions of the United States are well represented, but fewer compliance monitoring data sets are available for the States in the New England, Mid-Atlantic, and Southeastern regions.
These compliance monitoring data sets offer several benefits. For many States, they represent almost every groundwater and surface water community water supply (CWS) system in the State. In total, the compliance monitoring data in these databases represent over 18,000 of the approximately 24,000 CWS systems that do not purchase water in the United States; there are a total of about 54,000 CWS systems in the United States. In addition, a smaller number of nontransient, non-community water supply (NTNCWS) systems are also represented in the State compliance monitoring data sets. These data sets contain multiple samples from individual systems, which can facilitate analysis of the variability in arsenic levels over time, or from location to location, or point-of-entry to point-of-entry, within individual systems.
Since these scales on pipes and other components in distribution systems may contain relatively high arsenic concentrations, in some cases consumer tap water may exceed the drinking water standard for arsenic and become a health threat to consumers. But the truth is until there is new data from the water utility community, the relative amounts of arsenic perhaps being drunk by the population is not known.